By Paul Roggemans, Damir Šegon, Denis Vida, John Greaves, Takashi Sekiguchi, Alex Angelsky and Alexander Davydov
Abstract: An outburst near the anti-helion source has been registered by the cameras of the Global Meteor Network on 2022 August 15–16 and August 16–17. The shower meteors radiated from a very compact radiant centered at α = 325.3 ± 0.4° an δ = –11.5 ± 0.4° with a geocentric velocity vg = 23.9 ± 0.3 km/s, with M2022-Q1 as temporary identification, likely recorded before as the August delta Capricornids (ADC#00199). The flux plot indicates that the activity lasted ~15 hours with the main peak activity at λʘ = 143.71° corresponding to 2022 August 16, 22h04m UT with a ZHR of about 10. The mean orbit could be derived from 123 very similar orbits. Another set of 5 paired meteors recorded by the RMS network in Ukraine confirms the orbit obtained by GMN. The observed outburst matches very well with the forecast by Mikhail Maslov who predicted that a young trail of comet 45P/Honda-Mrkos-Pajdusakova ejected in 1980 could encounter the Earth at 2022 August 16, at 23h40m UT.
Introduction
On 2022 August 17, amateur astronomer Ivan Sergei from Belarus sent me the following message: “I just saw a concentration of an unclassified radiant near Capricorn with RA~21:42 DEC ~ –9 (lamda 179.8, beta 2.4 deg, Sol.long ~143.2), vg ~ 24 km/s according to the CAMS video network. Perhaps a new meteor shower is emerging”, adding a screenshot of the CAMS website (Figure 1).
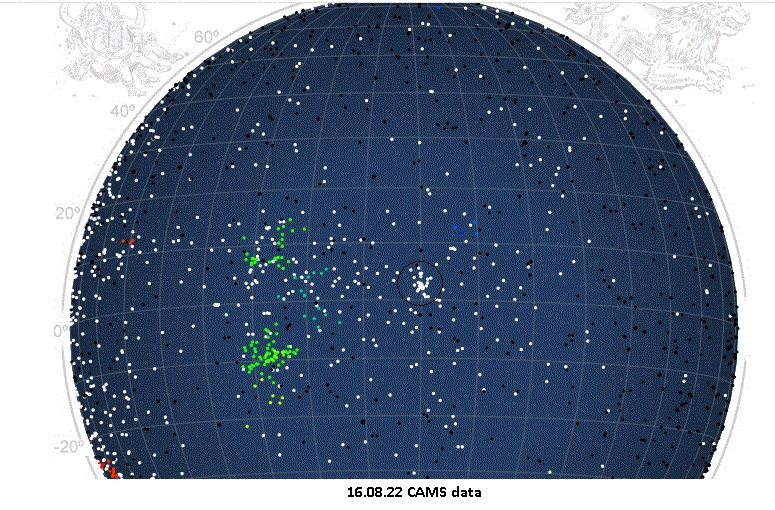
Figure – 1 Screenshot of the CAMS radiant plot that caught the attention of Ivan Sergei.
At that time Peter Jenniskens already had a CBET ready (Jenniskens, 2022a) to announce the shower outburst and the same day a report was published on MeteorNews (Jenniskens, 2022b). Already the next day a confirmation followed by the Japanese SonotaCo network (Sekiguchi, 2022).
The night of the outburst, August 16–17, much of Europe had a partially cloudy night after a long period with stable clear nights. Clouds hampered registration of meteors at many GMN camera stations but still a fair number of orbits could be recorded (Figure 2).
A planned migration of the server with the GMN data caused some delay in the processing of the new incoming data, but also the Global Meteor Network detected the outburst as a very compact radiant source (Figure 3).
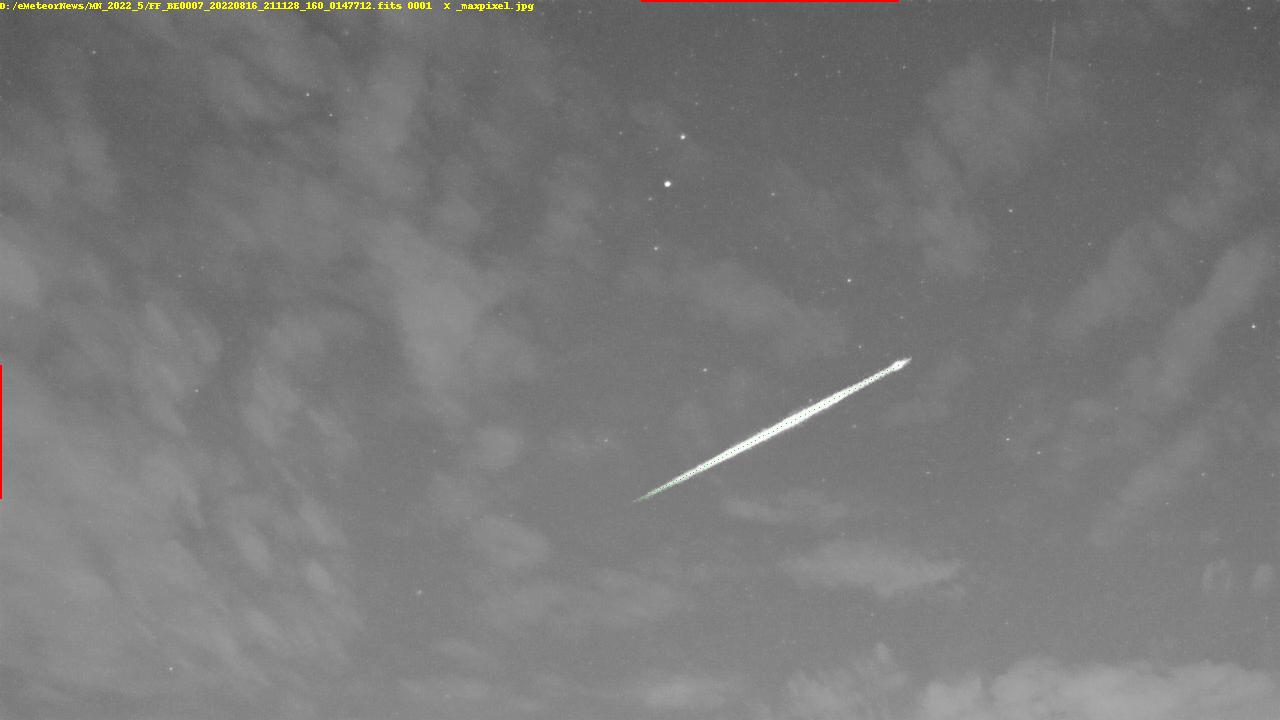
Figure 2 – A –2 meteor of this meteor outburst at 2022 August 16, 21h11m30s UT recorded by the GMN camera BE0007 in Genk, Belgium, paired with DE0005 and FR0006.
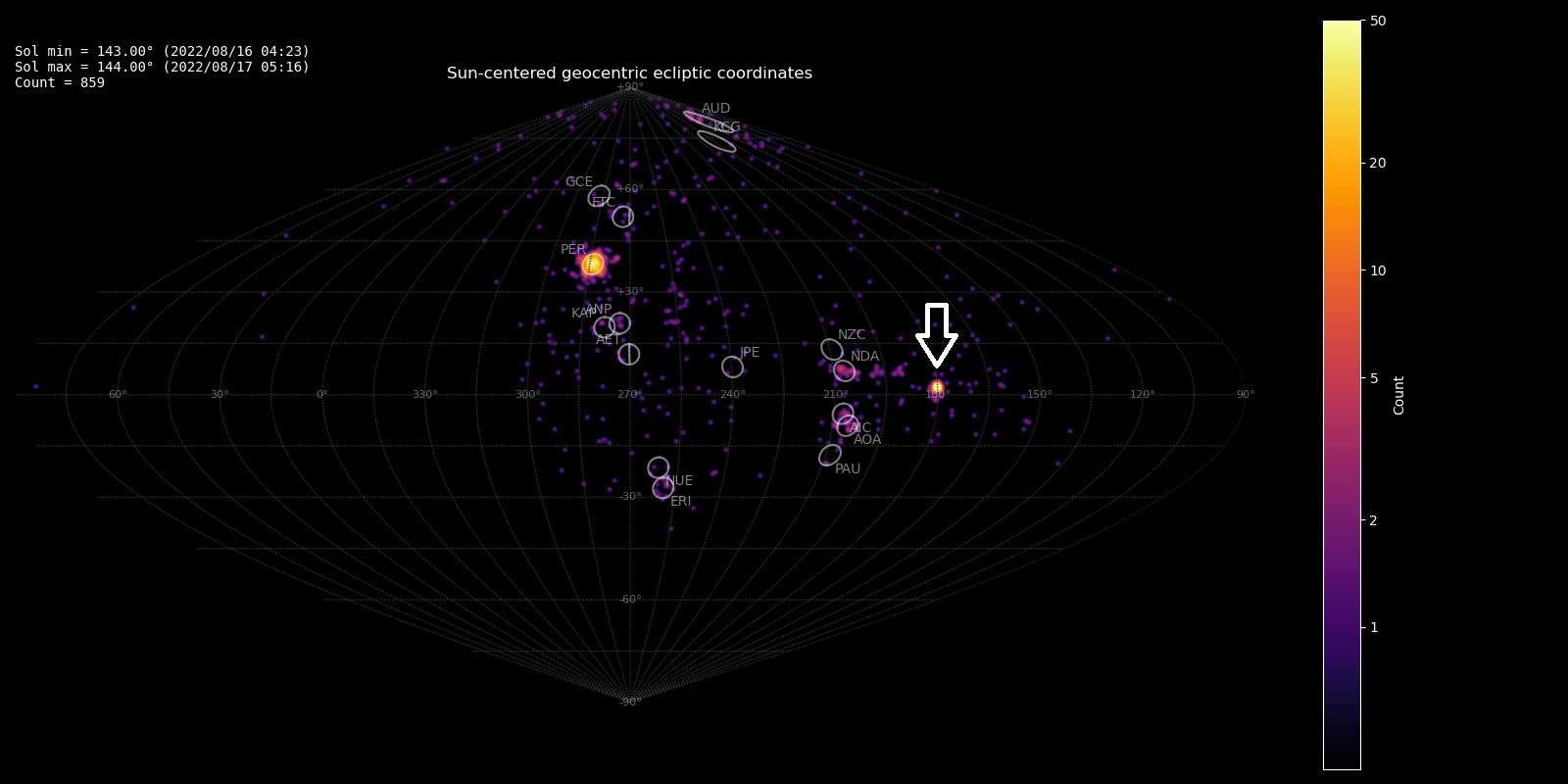
Figure 3 – The radiant density plot in Sun-centered geocentric ecliptic coordinates for the night August 16–17, 143°< λʘ <144°.
Global Meteor Network data
When the data was processed for the suspect period, an extract was downloaded with all GMN orbits obtained after λʘ = 142° (2022, August 15,03h23m04s UT) until the latest data available at the time of the download, λʘ = 144.772° (2022 August 18, 00h35m34s UT). This dataset includes 2307 orbits and is publicly available for download from the GMN website.
The median values for the orbit published by Jenniskens (2022a; 2022b) were used as initial reference orbit to start an iterative search for the best fitting mean orbit for a concentration of similar orbits. The method used for this has been described before (Roggemans et al., 2019) and combines three classic discrimination criteria, considering different classes for the degree of similarity. The discrimination criteria used in this method are that of Southworth and Hawkins (1963), identified as DSH, Drummond (1981), identified as DD, and Jopek (1993), identified as DH. The method to compute the mean orbit during the iteration process has been described by Jopek et al. (2006).
The position of the radiant of the outburst near the anti-helion source is a tricky region to look for orbit similarity because that area is full of sporadic Jupiter-family comets’ orbits with 2.0 ≤ TJ < 3.0. This means there is a high risk for contamination with sporadic orbits that fit the similarity criteria by pure chance. In such a case it depends on the compactness of the outburst to distinguish orbits related to the outburst from the rich sporadic background. In this case the iteration procedure converted very quickly after few steps at a best fitting mean orbit for 123 very similar orbits with DSH < 0.05, DD < 0.02 and DH < 0.05.
GMN results
The median value for the geocentric radiant position is α = 325.3 ± 0.4° and δ = –11.5 ± 0.4°, with vg = 23.9 ± 0.3 km/s, derived from the 123 most similar orbits obtained. Figure 4 shows the radiant plot color coded for the different thresholds of similarity. If we consider the most tolerant criteria with DSH < 0.25, DD < 0.105 and DH < 0.25, 169 orbits fit this criterion (blue dots). Using DSH < 0.10, DD < 0.04 and DH < 0.10, still 139 orbits can be accepted (red dots). However, considering the risk for sporadic contamination near the anti-helion source which is rich in JFC meteor orbits, we focus on the strictest discrimination threshold with DSH < 0.05, DD < 0.02 and DH < 0.05, visible in Figure 4 as the dense concentration of yellow dots. The lower threshold points marked in blue, green and orange appear very dispersed and are likely sporadics that fit the criteria by pure chance.
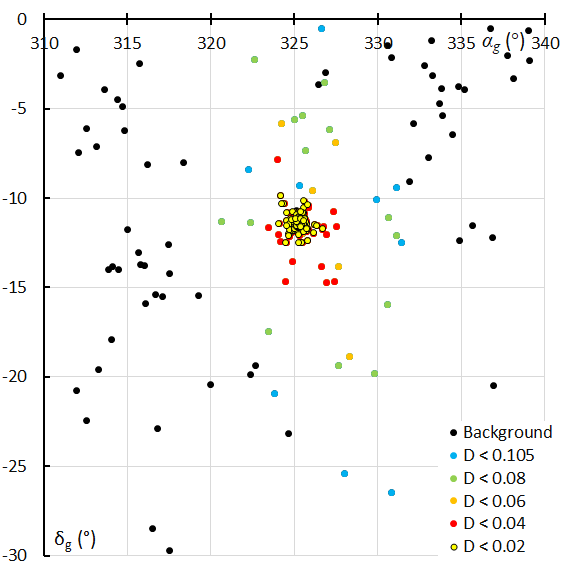
Figure 4 – The geocentric radiants in equatorial coordinates.
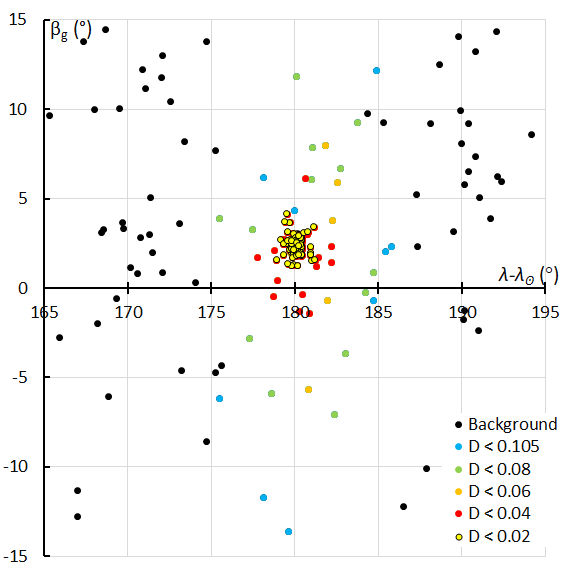
Figure 5 – The geocentric radiants in Sun-centered ecliptic coordinates.
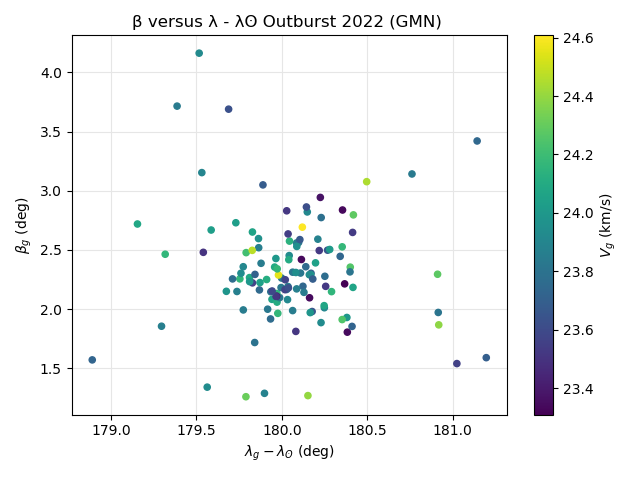
Figure 6 – The geocentric radiants in Sun-centered ecliptic coordinates, color coded for velocity.
Looking at the Sun-centered geocentric ecliptic radiant coordinates we see the same very dense concentration of radiant points (Figure 5). Within the small size radiant in Sun centered geocentric ecliptic coordinates color coded for velocity, we see no trend in variation in velocity (Figure 6). Checking if we find any trace of activity in past years, we applied the same stream search on all available orbits in the period 2019–2021 within the interval 142° < λʘ < 144.772°, a dataset with 6024 orbits. The result is shown in Figure 7, no concentration is found. The orbits that fit the discrimination criteria in previous years are likely sporadic orbits that fulfill the criteria by pure chance. Removing these from Figure 7 would create an empty space in the plot.
Trying to establish the time of peak activity we compare the number of orbits associated with the outburst as a percentage relative to the number of orbits not related to the outburst, or the so-called background activity. We did not filter other meteor showers as these are relative stable during the short activity period. To avoid too small number statistics, we left out the time intervals with too few data. Unfavorable weather and poor coverage at some longitudes caused the gaps in the activity profile. Luckily the main peak activity occurred over Europe where the best coverage of GMN is situated. Figure 8 shows the activity profile with the different thresholds of similarity. The dispersed low threshold orbits (blue, green and orange) can be ignored, the most relevant activity was caused by the very similar orbits (red and yellow). Peak activity occurred at λʘ = 143.71° corresponding to 2022 August 16, 22h04m UT. The total activity period covered about 24 hours with the first orbits being detected in the night of August 15–16. After the peak, the activity faded out within about 5 hours. Both the small radiant size and the narrow activity profile indicate an encounter with a very compact dust cloud. The flux plot is shown in Figure 9.
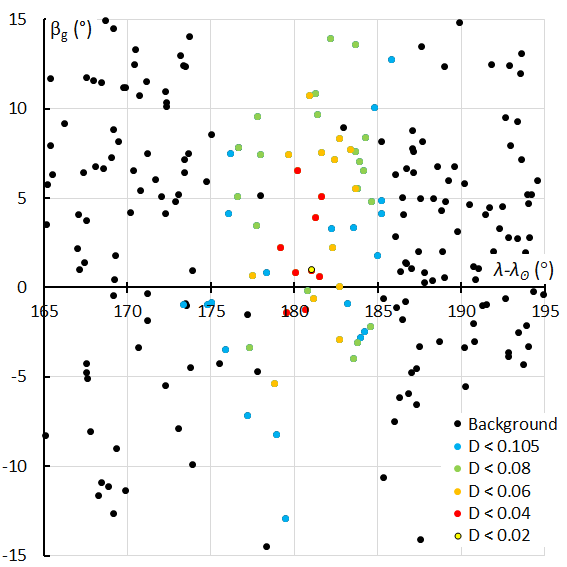
Figure 7 – Same plot as Figure 5 but with GMN data from 2019, 2020 and 2021.
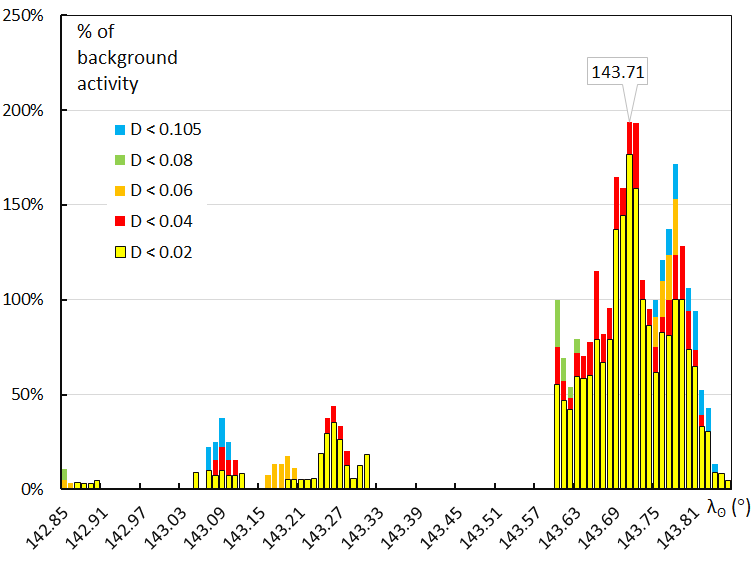
Figure 8 – The activity profile with the number of orbits caused by the outburst as a percentage relative to the number of orbits not associated with the outburst.
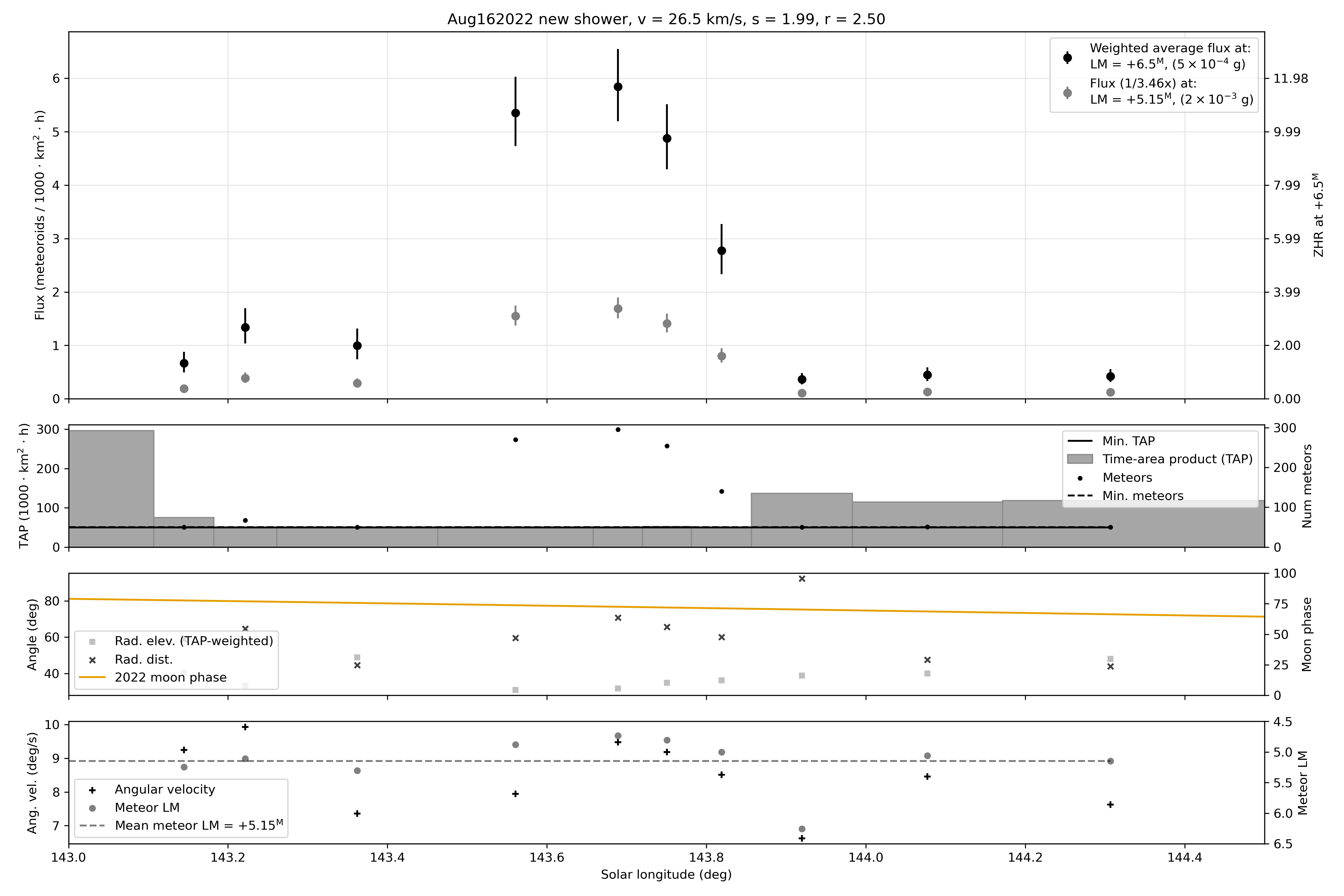
Figure 9 – The flux plot assuming a standard value of s = 2.0, the ZHR is about 10. The activity lasted only ~15 hours.
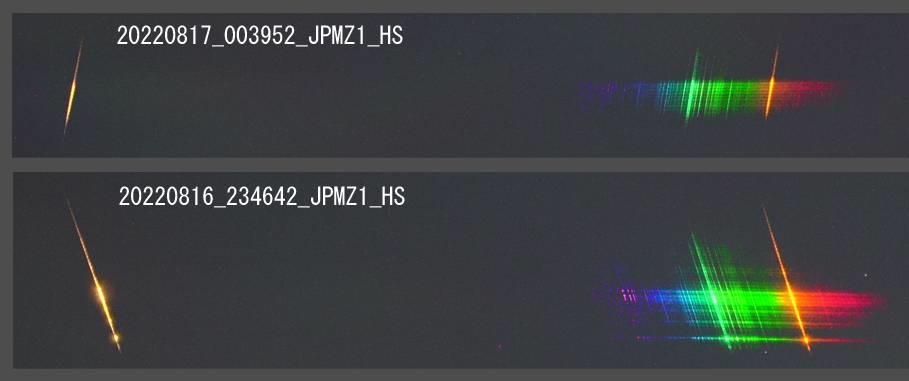
Figure 10 – Spectrum images of new shower members, α7s, 35mm, 600 gratings reduced to 4K30p ½, dispersion direction corrected. (SonotaCo Network, Japan, recording by Maeda).
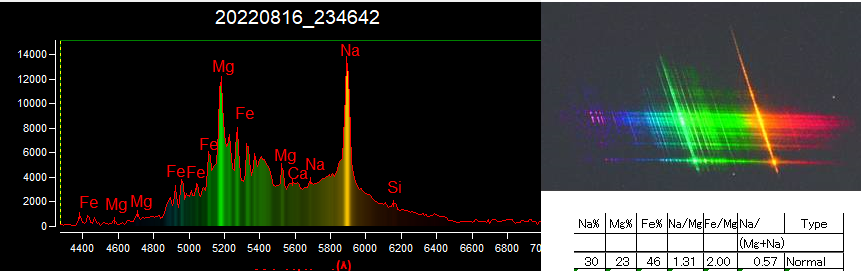
Figure 11 – Results of the spectral analysis of the new meteor shower with the identification of the emission lines, ratio of the emission lines and the type of classification.
The SonotaCo network in Japan obtained spectral data of meteors belonging to the new shower (Figure 10). The spectral analysis shows a normal type of spectrum rich in Iron (Fe 46%), Natrium (Na 30%) and Magnesium (Mg 23%) (Figure 11).
Table 1 – Comparing the orbit parameters obtained by GMN with those of CAMS (Jenniskens, 2022c) and SonotaCo.
GMN | CAMS | SonotaCo | |
α (°) | 325.3 ± 0.4 | 325.28 ± 0.06 | 324.9 ± 0.04 |
δ (°) | –11.5 ± 0.4 | –11.40 ± 0.06 | –11.9 ± 0.3° |
vg (km/s) | 23.9 ± 0.3 | 24.12 ± 0.14 | 23.9 ± 0.5 |
Hb | 98.1 ± 2.6 | – | 98.4 |
He | 84.0 ± 4.2 | – | 79.9 |
λ–λʘ (°) | 180.04 ± 0.35 | – | – |
β (°) | +2.28 ± 0.45 | – | – |
a (AU) | 2.91 ± 0.15 | 3.16 | 3.03 |
q (AU) | 0.551 ± 0.005 | 0.547 ± 0.025 | 0.554 ± 0.005 |
e | 0.811 ± 0.01 | 0.823 ± 0.069 | 0.816 ± 0.015 |
i (°) | 1.81 ± 0.36 | 1.90 ± 1.30 | 1.61 ±0.19 |
ω (°) | 270.9 ± 0.7 | 270.7 ± 2.3 | 270.5 ± 0.12 |
Ω (°) | 143.75 ± 0.18 | 143.2 ± 0.7 | 143.4 ± 0.15 |
Π (°) | 54.6 ± 0.7 | – | – |
Tj | 2.66 ± 0.09 | – | – |
P (Y) | 4.98 ± 0.39 | – | – |
N | 123 | 137 | 4 |
The mean orbit (Jopek et al., 2006) for this new shower has been calculated using only orbits with DSH < 0.05, DD < 0.02 and DH < 0.05. The result based on 123 orbits is listed in Table 1 and compared with the results obtained by CAMS and SonotaCo.
The close-up of the orbit distribution with inclination i plotted against longitude of perihelion Π shows no trend in the velocity distribution. The small variation in velocity is within the error margins of the measured velocities
(Figure 12).
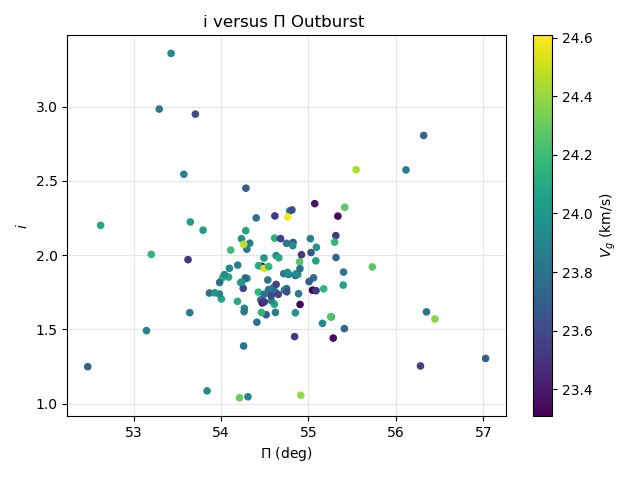
Figure 12 – Concentration of orbits with the inclination i plotted against the longitude of perihelion Π, color coded for velocity.
The distributions of the orbital elements plotted in Figures 13, 14 and 15, show the dense concentration of the 123 orbits used to compute the mean orbit (yellow dots). The more dispersed orbits marked in blue, green and orange may be likely sporadic orbits of the anti-helion source which accidently fit the similarity criteria. The dispersion of these
orbits compared to the dense concentration (yellow) is also obvious in the histograms with the distributions of the eccentricity e, perihelion distance q, inclination i and the longitude of perihelion Π (Figures 16, 17, 18 and 19). The variation in geocentric velocity vg varies within the measurement accuracy interval (Figure 20).
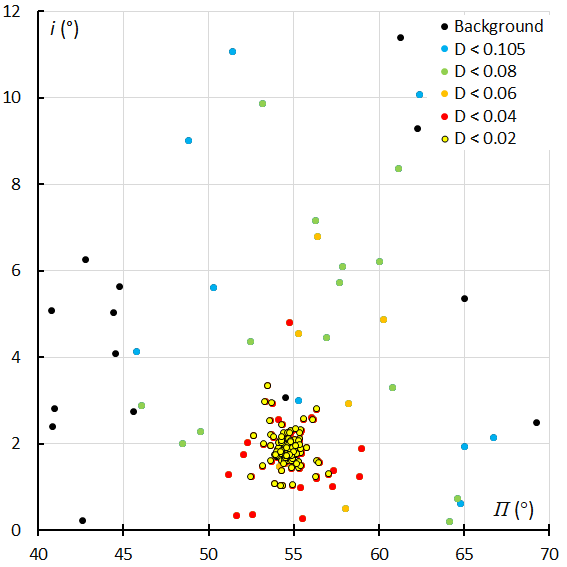
Figure 13 – Concentration of orbits with the inclination i plotted against the longitude of perihelion Π.
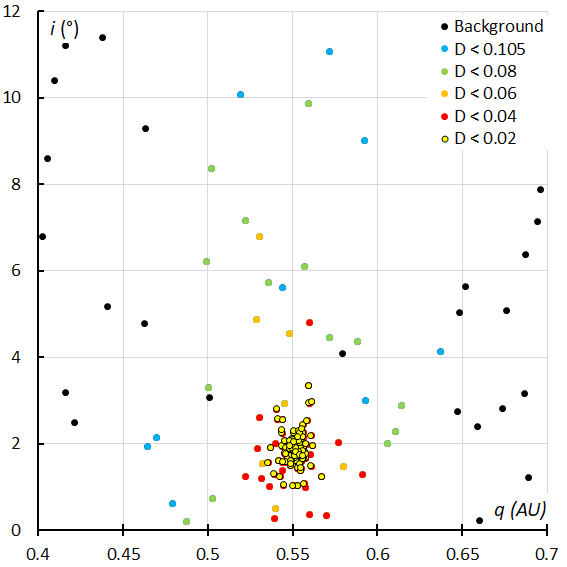
Figure 14 – Concentration of orbits with the inclination i plotted against the perihelion distance q.
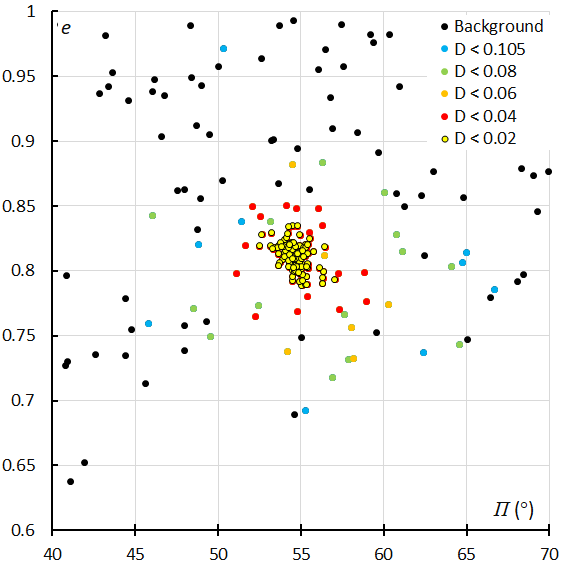
Figure 15 – Concentration of orbits with the eccentricity e plotted against the longitude of perihelion Π.
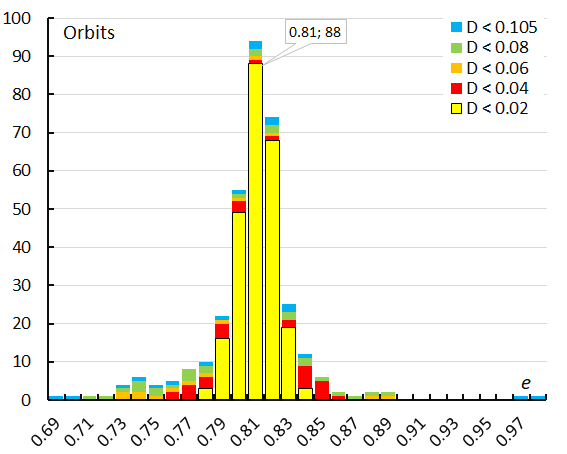
Figure 16 – The histogram with the distribution of the eccentricity e showing the spread for the different thresholds of similarity.
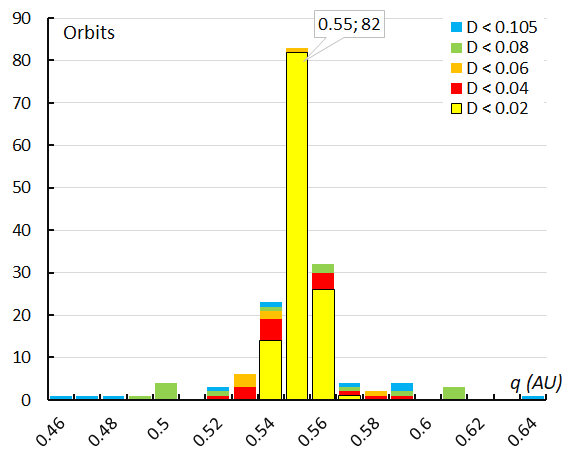
Figure 17 – The histogram with the distribution of the perihelion distance q showing the spread for the different thresholds of similarity.
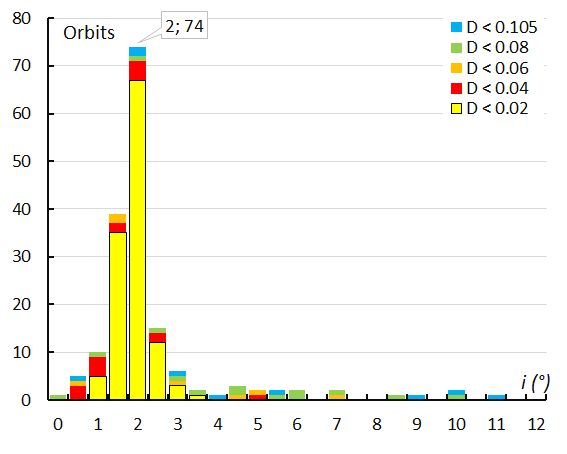
Figure 18 – The histogram with the distribution of the inclination i showing the spread for the different thresholds of similarity.
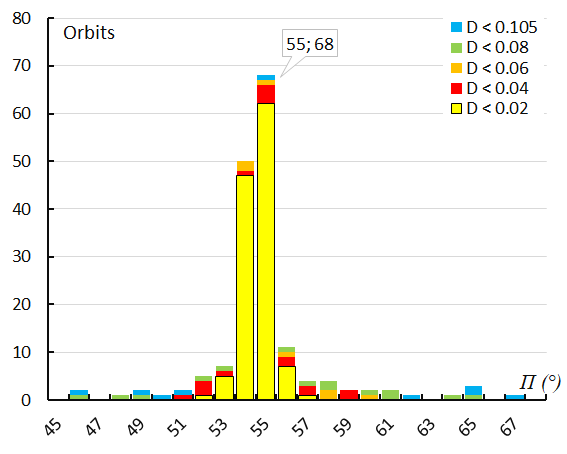
Figure 19 – The histogram with the distribution of the longitude of perihelion Π showing the spread for the different thresholds of similarity.
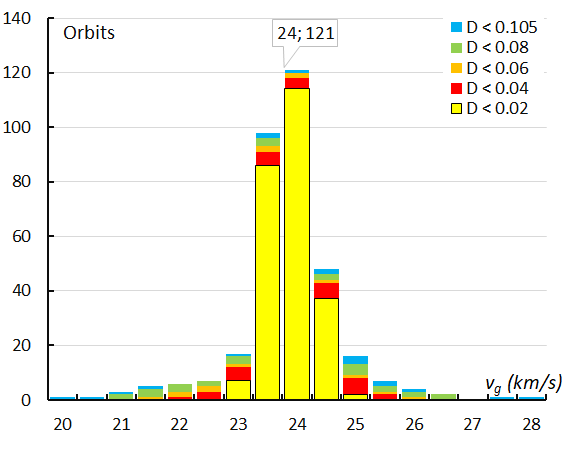
Figure 20 – The histogram with the distribution of the geocentric velocity vg showing the spread for the different thresholds of similarity.
Confirmation by the Ukrainian RMS network
Despite the ongoing war, observers from Ukraine independently got the same results based on 5 paired meteors recorded by RMS cameras at the Ukrainian camera stations in the southwest of Ukraine. The mean orbit has been calculated using the method of Jopek et al. (2006). The Ukrainian results are listed in Table 2 and in excellent agreement with the GMN, CAMS and SonotaCo results listed in Table 1. Figures 21 and 22 display two meteors from the outburst. Figure 23 shows a plot of the orbits.
Table 2 – The Orbit parameters obtained by the RMS network in Ukraine.
α (°) | 325.6 ± 0.2 |
δ (°) | –11.6 ± 0.4 |
vg (km/s) | 23.3 ± 0.3 |
Hb | 98.7 ± 2.5 |
He | 83.6 ± 2.7 |
a (AU) | 2.65 ± 0.13 |
q (AU) | 0.555 ± 0.003 |
e | 0.791 ± 0.01 |
i (°) | 1.53 ± 0.36 |
ω (°) | 271.3 ± 0.3 |
Ω (°) | 143.76 ± 0.04 |
N | 5 |
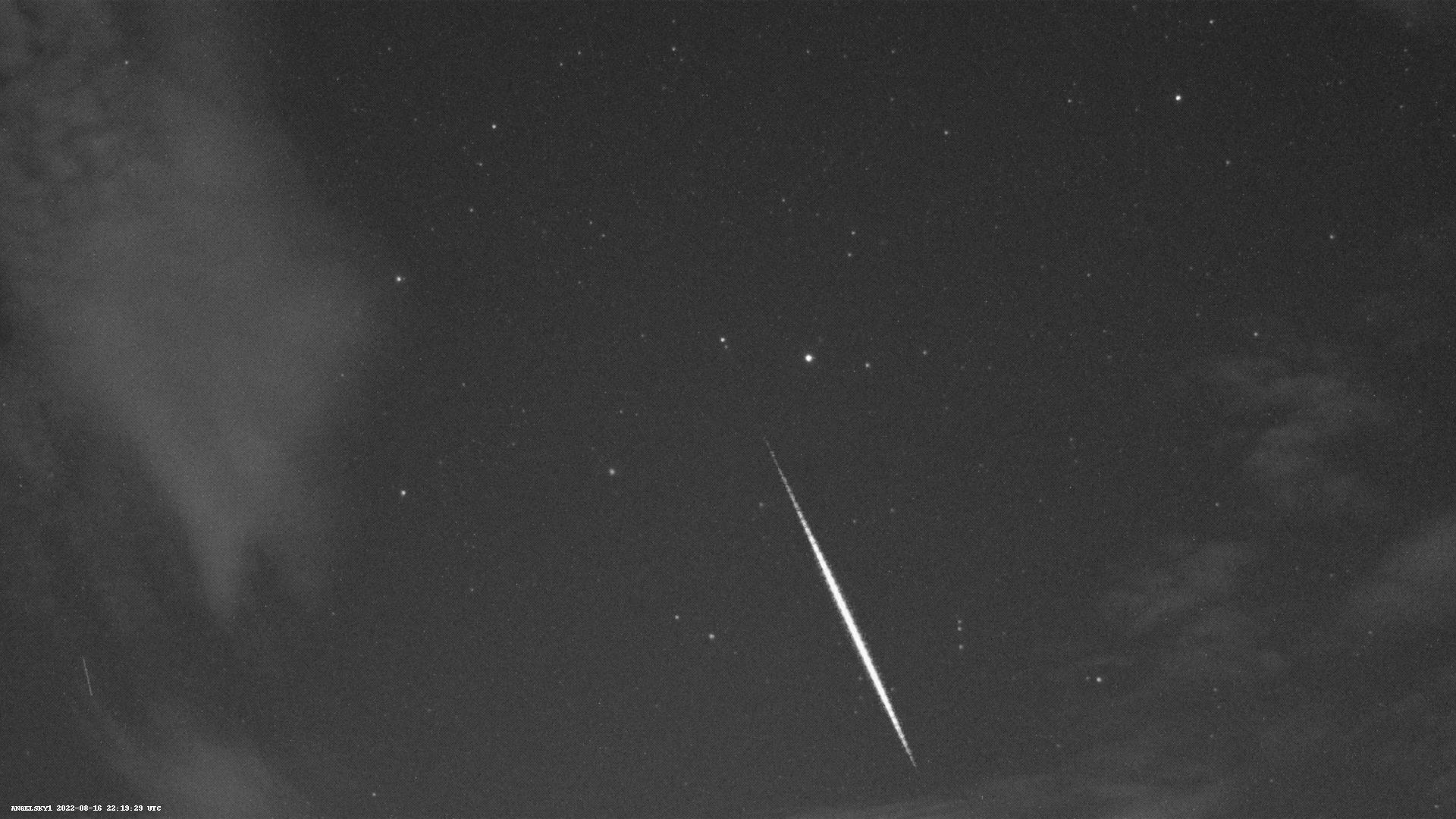
Figure 21 – Shower member recorded 2022 August 16, 22h19m29s UT, at Odessa, Ukraine (photo Alex Angelsky).
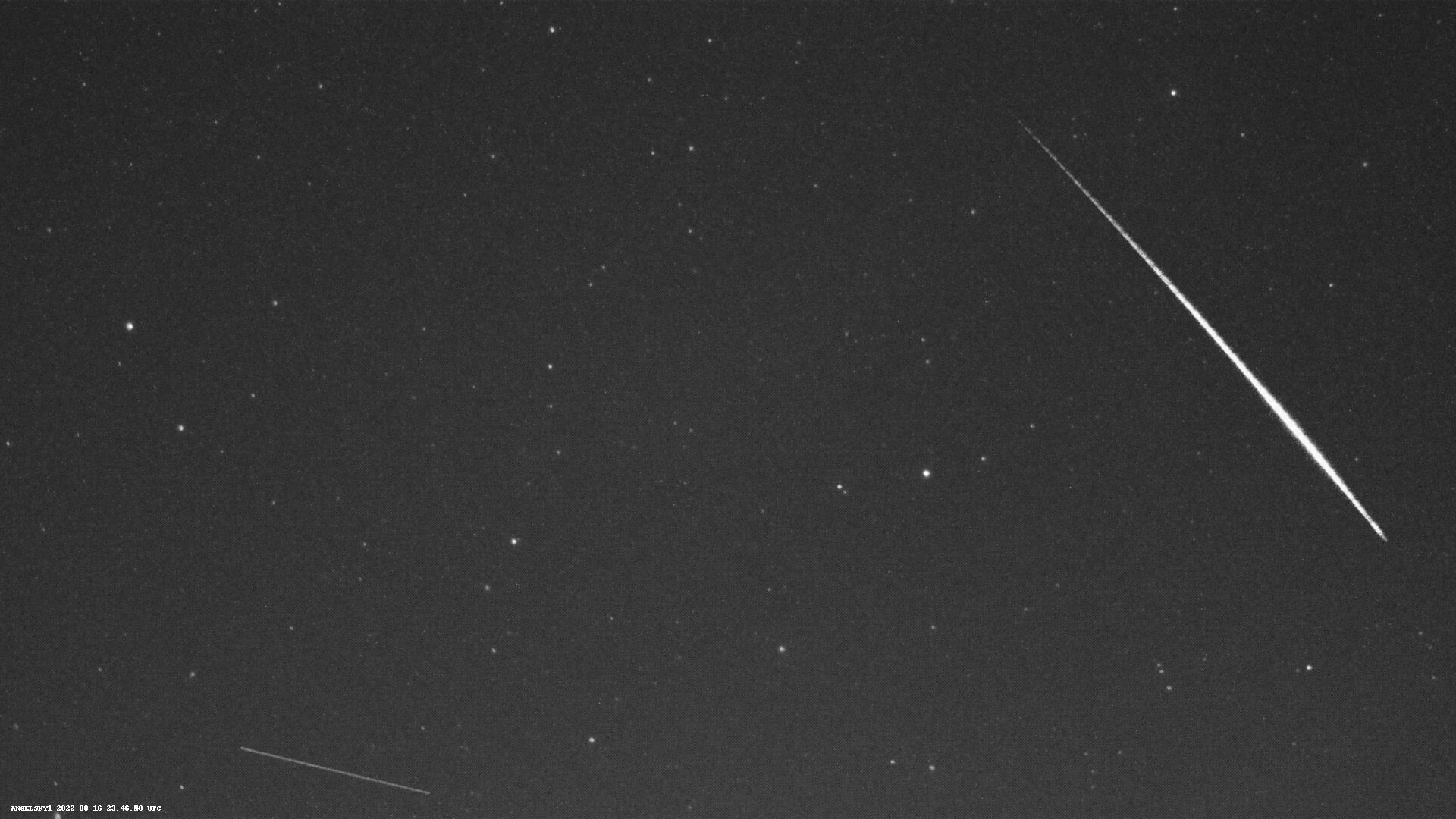
Figure 22 – Shower member recorded 2022 August 16, 23h46m48s, at Odessa, Ukraine (photo Alex Angelsky).
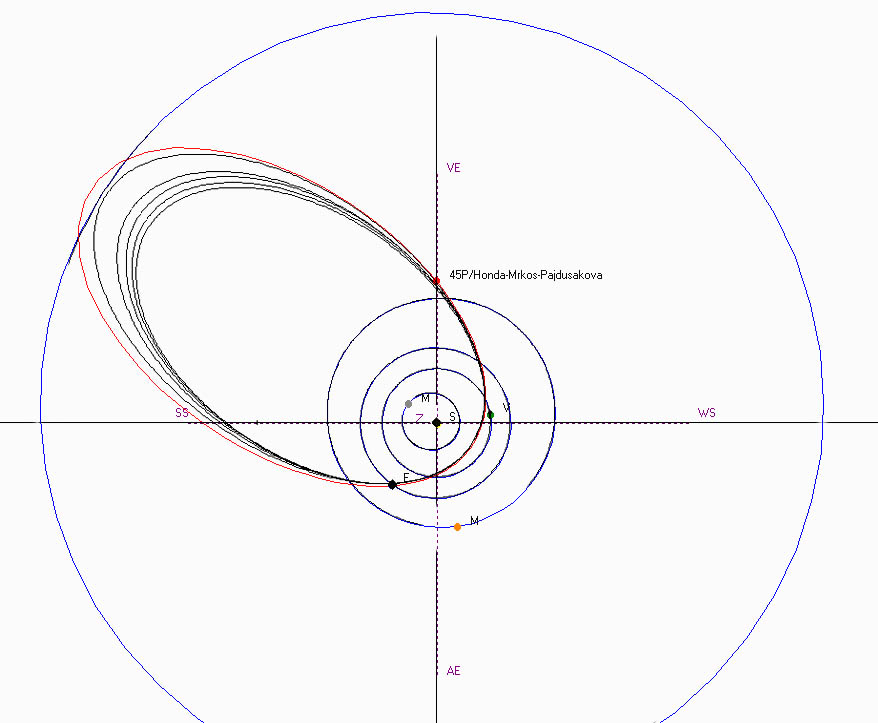
Figure 23 – The orbit of the parent body (red) and the 5 orbits recorded by the Ukrainian network.
New or already known shower?
In the IAU MDC meteor shower list, we find an entry listed as the August delta Capricornids (ADC#00199) associated with parent body 45P/Honda-Mrkos-Pajdusakova, active at λʘ = 146°, with a radiant at α = 328.7° and δ = –16°, vg = 21.6 km/s with orbital elements a = 2.414 AU, q = 0.597 AU, e = 0.753, i = 2.8°, ω = 87.3° and Ω = 327°, based on as few as 6 single orbits. The radiant is just south of the ecliptic in ecliptic latitude while our outburst radiant is just north of the ecliptic in ecliptic latitude.
This entry is based on a stream search on a set of over 1000 photographic fireball orbits of meteors brighter than magnitude –3 using only the Southworth and Hawkins criteria (Porubčan and Gavajdová, 1994). The photographic fireball data includes all-sky camera data which in general is less accurate and unsuitable for orbit determination apart from some properly investigated meteorite droppers. It is not clear which threshold has been used to avoid contamination by sporadics fitting the criteria by pure chance. The orbit listed for ADC#00199 should be regarded with caution as we do not know how it was obtained. Unfortunately, this cannot be verified anymore and we do not know the reliability of the fireball data used.
Parent body
Japanese observers concluded that the orbit of the new meteor shower resembled the low inclination orbit of comet 45P/Honda-Mrkos-Pajdusakova which is the most likely parent body responsible for the encounter with this dense dust cloud.
Accordingly orbits for 45P for the discovery apparition of 1948 onward were obtained from the IAU Minor Planet Center and tested against the GMN orbit listed in Table 1 using the DH criterion (Jopek, 1993) with a detection threshold of 0.10. An interaction with Jupiter at a closest distance of 0.11 AU (Astronomical Units) on 1983 March 26th decreased the comet’s perihelion distance from roughly 0.58 to 0.54 AU and its inclination from around 13 to 4 degrees, as well as changes in other orbital elements, such that after the 1980 apparition the following apparitions would have somewhat different orbital parameters, as can be seen in the IAU MPC data. This would also likely include modifications to the orbits of any material ejected during the 1980 apparition and perihelion. Since that time the orbit has been more favorable to generating meteor streams impinging on Earth’s orbit, and indeed the parent body itself has made relatively close approaches to Earth in 2011 and 2017. It will again return to a larger perihelion distance and inclination amongst other orbital element changes after a 0.17 AU Jupiter encounter in 2030 (e.g., Kinoshita, Kazuo), although it should be noted that future orbit predictions are complicated by this comet having variable non-gravitational factors.
Table 3 – The criteria DSH, DD and DH for the GMN orbit in comparison to the epoch year for the orbit of each perihelion passage of 45P from 1948 to 2032 are given with threshold limits of 0.15, 0.10 and 0.10 respectively. Any value in the table below that number can be considered a valid association, the lower the better, whilst any value above it cannot.
45P year | DSH | DD | DH |
1948 | 0.235 | 0.076 | 0.235 |
1954 | 0.236 | 0.076 | 0.236 |
1964 | 0.236 | 0.076 | 0.236 |
1969 | 0.236 | 0.076 | 0.236 |
1974 | 0.237 | 0.079 | 0.237 |
1980 | 0.237 | 0.08 | 0.237 |
1985 | 0.063 | 0.022 | 0.063 |
1990 | 0.063 | 0.023 | 0.063 |
1995 | 0.066 | 0.028 | 0.066 |
2001 | 0.068 | 0.03 | 0.067 |
2006 | 0.067 | 0.029 | 0.067 |
2011 | 0.067 | 0.029 | 0.067 |
2016 | 0.066 | 0.028 | 0.066 |
2022 | 0.066 | 0.022 | 0.066 |
2027 | 0.066 | 0.022 | 0.066 |
2032 | 0.218 | 0.092 | 0.214 |
Taking this into consideration it should be noted that the orbits from 1948 to 1980 inclusive did not pass the DH < 0.1 threshold when tested against the GMN orbit, whilst the 1985 to current orbits do. The DSH, DD and DH values for the GMN orbit tested against the orbits for the comet’s apparitions from 1948 to 2032 are given in Table 3. Note that the standard threshold value for DD appears to be too generous for very low inclination objects relative to the other two criteria. It should be further noted that only these orbits for 45P passed this threshold mark when a selection of 1170 cometary orbits (inclusive of multiple apparition orbits for some periodic comets) having perihelion distance less than 1.2 AU, including D/1770 Lexell, were similarly tested against the GMN orbit for this shower. Not one other of these comets matched the GMN orbit to better than DH = 0.10, thus it can be taken from this that the Japanese team’s suggestion of 45P being the potential parent body for the current outburst, and that despite the tendency of Jupiter Family Comets to have similar orbits such that at times many comets can be matched to one shower, no other comet can be shown to be a potential parent body to the outburst being analyzed. It also appears that the changes in the orbit of the comet will make it a potential continuing source for Earth crossing meteoroids for only around 50 years.
Moreover, some years ago this outburst had been computed by Mikhail Maslov. He predicted: “On (2022) 16 August at 23h40m UT the Earth is expected to encounter the young trail of the comet 45P/Honda-Mrkos-Pajdusakova ejected in 1980. The parameters of the encounter are the following: minimum distance is 0.00377 AU, ejection velocity is 9.82 m/s, trail density is 373.2% of that for 1 rev. Leonid trail. While the computed minimum distance to the central axis of the 1980 trail is quite large, the rest encounter parameters (ejection velocity and trail density) are quite favorable. So far, some minor activity is possible around the given maximum time and observations for checking this forecast are recommended.”. It is obvious that this is what we encountered in the form of a very compact dust cloud, slightly earlier in time than predicted.
Conclusion
The Global Meteor Network has once again succeeded in achieving its objective, not to let any unexpected meteor shower pass unnoticed. A compact radiant centered at α = 325.3 ± 0.4° an δ = –11.5 ± 0.4°, has been recorded. This is close to the radiant predicted by Mikhail Maslov at α = 326.8°, δ = –15.1°. The peak occurred at λʘ = 143.71° or 2022 August 16, 22h04m UT, about 1.5 hours sooner than predicted with a ZHR of about 10. The outburst has been confirmed by the CAMS, SonotaCo and Ukrainian networks. The activity might have been strong enough to catch attention from visual observers.
Acknowledgement
The authors thank all the camera operators and people involved in the Global Meteor Network. Orbits for this shower outburst were obtained by GMN RMS cameras installed in: Australia, Belgium, Bulgaria, Canada, Croatia, France, Germany, Hungary, Israel, Italy, Poland, Russia, Slovakia, Slovenia, Spain, Switzerland, United Kingdom, and the United States. The Ukrainian RMS network provided independent evidence. The Global Meteor Network (GMN) data are released under the license (click here). This research has made use of data and/or services provided by the International Astronomical Union’s Minor Planet Center.
References
Drummond J. D. (1981). “A test of comet and meteor shower associations”. Icarus, 45, 545–553.
Jenniskens P. (2022a). “18-Aquariid meteor shower 2022”. CBET 5159, published 2022, August 17. Ed.: D. W. E. Green. Cambridge: Central Bureau for Astronomical Telegrams.
Jenniskens P. (2022b). “Ongoing outburst from a new radiant on Aquarius/Capricorn border”. eMetN, 7, in print.
Jenniskens P. (2022c). “August delta Capricornids meteor shower 2022”. eMetN, 7, in print.
Jopek T. J. (1993). “Remarks on the meteor orbital similarity D-criterion”. Icarus, 106, 603–607.
Jopek T. J., Rudawska R. and Pretka-Ziomek H. (2006). “Calculation of the mean orbit of a meteoroid stream”. Monthly Notices of the Royal Astronomical Society, 371, 1367–1372.
Porubčan V., Gavajdová M. (1994). “A search for fireball streams among photographic meteors”. Planetary and Space Science, 42, 151–155.
Roggemans P., Johannink C. and Cambell-Burns P. (2019). “October Ursae Majorids (OCU#333)”. eMetN, 4, 55–64.
Sekiguchi T. (2022). “New radiant on Aquarius/Capricorn border by the SonotaCo Network”. eMetN, 7, in print.
Southworth R. R. and Hawkins G. S. (1963). “Statistics of meteor streams”. Smithson. Contrib. Astrophys., 7, 261–286.